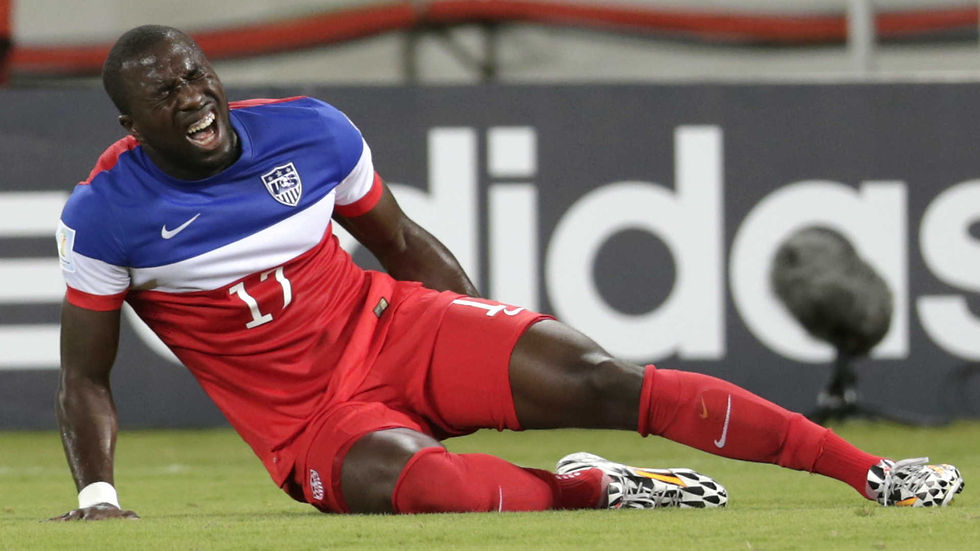
Similar to many other sports, playing football carries its own unique risk of injuries, many of which may in fact be preventable. One of the key roles of the strength and conditioning professional is to reduce injury occurrence, which involves minimising the risk of injury and keeping players in the competitive arena. Van Mechelen (1997) proposed a four-step model to follow when designing an injury prevention program that included i) establish the incidence of injury, ii) determine the mechanism of injury, iii) devise prevention program and finally iv) test the program by gathering new incidence data.
The incidence of injury in team sports such as football remains high with an elite team of 25 players expected to experience 50 injuries per season, half of them minor causing absences of less than a week but as many as eight or nine severe and causing absences of over 4 weeks (Ekstrand, Hagglund & Walden, 2009). Most football injuries affect the lower extremities, with the most common soft tissue injury occurring in the hamstring muscle in male players (Arnason et al, 2008) whereas non-contact anterior cruciate ligament tears seem to be more common in female players (Renstrom et al, 2008). The mechanism of hamstring injury is suggested to occur during the lateswing phase of running, where the muscle bulk reaches maximum elongation acting eccentrically to decelerate knee and hip extension in preparation for heel strike (Drezner, 2003). The high volume of accelerations and decelerations involved in intermittent sports such as football, exacerbates this aggressive eccentric hamstring activation, further increasing the risk of injury. In contrast, the two most commonly described ACL injury situations in football are turning of the trunk when the foot is fixed to the ground (rotation and abduction) or landing awkwardly from a jump (Faunø and Jakobsen, 2006). Such injuries can have numerous individual, social and economic consequences including extensive periods of nonparticipation as well as treatment and rehabilitation costs (Soomro et al, 2016). Injury risk factors can be classified as modifiable (e.g. strength, muscle imbalance, neuromuscular control/imbalance) or non-modifiable (e.g. age, gender, environment). With this in mind it is the modifiable risk factors that become of greater importance, as these can potentially be addressed through physical training such as strength, balance and control (Bahr, 2003).
Isokinetic dynamometers are devices used for both the measurement and conditioning of muscular strength under conditions of constant angular velocity. The maximum torque during isokinetic movements is a measure of the muscular force produced by all structures around a joint (both muscle and ligament) and is quantified as joint moment (Nm). Peak torque is an indication of the maximum strength that can be exerted at a given angular velocity, whereas the angle at which peak toque occurs highlights the angle at which the joint can produce the greatest amount of strength according to the length tension relationship. Peak torque values of the dominant and non-dominant legs can be used to identify strength asymmetries which could be a risk for injury however, peak torque asymmetries of up to 15% are considered acceptable. The conventional hamstring/quadriceps ratio (Hcon:Qcon) has traditionally been calculated as maximum knee flexion strength divided by maximal knee extension strength at a given angular velocity, through concentric muscle action of both hamstring and quadricep muscles (Crosier et al, 2008). With this in mind, Cameron et al (2003) suggested that a conventional Hcon:Qconof <0.66 significantly increased the risk of hamstring injury. Conceptually, the conventional Hcon:Qconratio implies that concentric contraction of the knee flexors or extensors would occur at the same time. However, natural joint movement only permit’s eccentric hamstring contraction to be coupled with concentric quadriceps contraction, or vice versa. For this reason, a more functional ratio of Hecc:Qconhas been suggested which better describes the agonist-antagonist relationship between knee flexion and extension. Croisier et al (2002) suggested a mixed (variety of velocities) functional ratio threshold of <0.80 to be indicative to injury risk, while Aagaard et al (1995) demonstrated the potential for a functional ratio of 1.0 showing that the braking action of the hamstrings to equal the maximal knee extension moment. The specific limitations of this test include a lack of ecological validity to real sporting movements due to its open kinetic chain nature, and also that physiological movement may not be simulated during constant velocity limb movements (Gleeson and Mercer, 1996). Additionally, the velocity of movement still does not reflect the actual speed of movement that the athlete experiences during sporting performance.
There are multiple factors that may influence the risk of ACL injury in male and female athletes, although neuromuscular control may be the most important and modifiable factor that can be addressed. Hewett et al (1996) looked at the neuromuscular control of knee biomechanics during sport actions and found that peak landing forces were significantly predicted by knee valgus torque, decreased relative knee flexor torque when landing and hamstring peak torque imbalances. Neuromuscular imbalances that occur during high dynamic loading of the knee along with measures of external knee abduction moment during landing tasks may be important contributors to ACL injury risk (Bien, 2011). It has been suggested that the tuck jump test may be useful in identifying extremity landing technique flaws during plyometric activity, as increased valgus knee alignment during jump landing has been demonstrated as a risk factor in ACL injury (Boling et al, 2009).
With these concerns in mind identifying players that may be in need of addressing these modifiable risk factors may go a long way in preventing injury occurrence. Therefore, this case study will analyse and interpret data collected from isokinetic dynamometry testing along with a basic field test (the tuck jump test) to help guide the design of an injury prevention program for a football player.
Isokinetic Dynamometry
Method
The test can be administered in either a seated or prone position. For ease and efficiency both eccentric and concentric testing was performed in the seated position. The athlete was strapped into seat of the machine in the upright position. The athletes knee joint is aligned with the axis of rotation of the dynamometer, and the limb being tested is attached to the lever arm of the isokinetic device. During measurement of concentric strength, the athlete is instructed to push and pull as hard and fast as possible against the lever arm; during eccentric testing, the athlete resists the movement as much as possible. The dynamometer resists the motion as needed to maintain a constant pre-set velocity. Concentric strength was measured at 60˚/sec and 120˚/sec in flexion and extension, whereas eccentric strength was measured at 30˚/sec and 60˚/sec in extension only.
Results
Table 1 and 2 show the absolute isokinetic concentric and eccentric peak torque during flexion and extension of the knee for both the dominant and non-dominant legs. Peak torque decreased as movement speed increased, as higher movement velocities reduce the time available for cross bridge interaction. There is an imbalance observed between the concentric extension action of the dominant and non-dominant leg at 60˚/sec during flexion and at 120˚/sec during extension; in both conditions, the dominant leg appears stronger. However, they both fall within the 15% imbalance range; it should also be recognised that it is in fact higher levels of eccentric strength and not concentric strength that has been associated with reduced hamstring injury risk (Fousekis et al, 2011). The angular position is important as it provides an indication of muscle function by offering information about the mechanical properties of the contracting muscle (Baltzopoulos and Brodie, 1989). Peak torque localised at a more open knee angle (figure 1 and 2) has been suggested to be beneficial in hamstring injury prevention (Bourne et al, 2017) as this increases the ability of the hamstrings to generate high levels of torque at longer muscle lengths. During the test, 0˚ indicates full extension therefore this athletes’ optimum peak torque angle occurs at 26˚and 35˚ (dominant and non-dominant leg) during flexion at 60˚/sec and 62˚ and 42˚ at 120˚/sec, indicating a relatively open angle at slower speeds, which is acceptable.
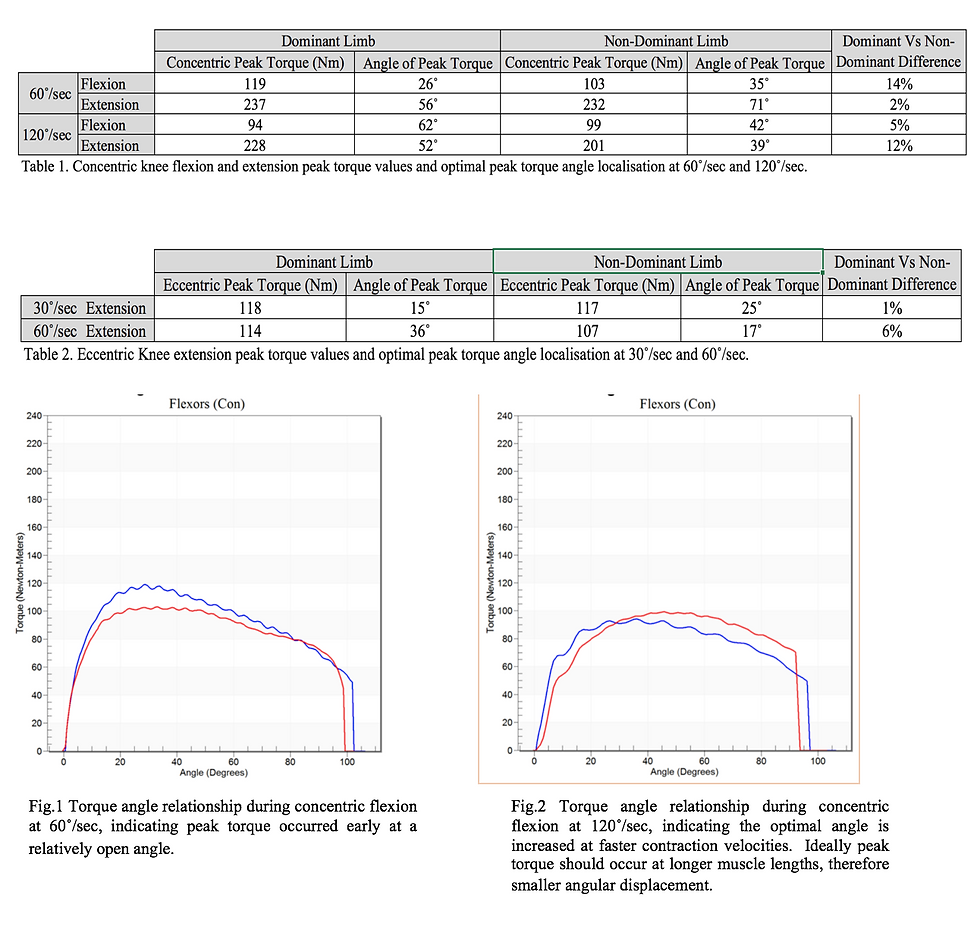
Table 3 and 4 show the results of the conventional and functional H:Q ratios. It has been suggested that H:Q ratios are more important than peak torque as it provides an indication of the muscular balance around a joint (Campbell and Glenn, 1982). Table 3 showsthat this athlete was unable to meet the threshold of 0.66 in either dominant or non-dominant legs, at both 60˚/sec and 120˚/sec. Table 4 shows that this athlete falls below this criteria at all functional ratio velocities. This imbalance suggests that there is insufficient eccentric braking capacity of the hamstring muscles compared to the concentric action of the quadriceps, which can place this athlete at a high risk of injury. An intervention to increase the eccentric strength of the hamstrings is therefore recommended for this athlete.
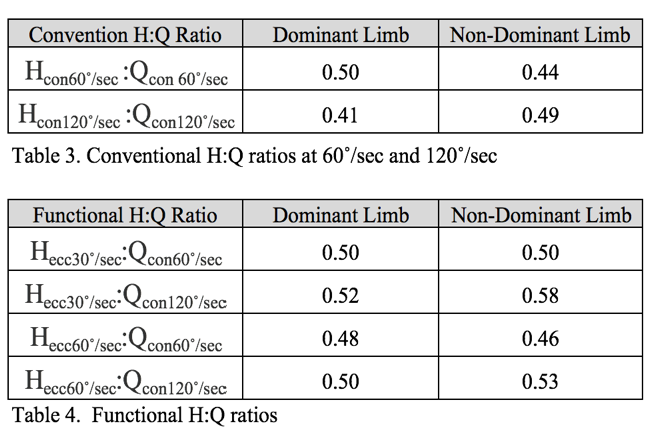
Recommendations
Increase eccentric strength of the hamstrings – increase functional ratio to >0.80
Askling et al (2002) observed an increase in both eccentric and concentric knee flexor strength after 10 weeks of eccentric YoYo flywheel training on a leg curl ergometer. The working principle behind this device is that the athlete sets the flywheel in motion with a concentric contraction, the athlete is then forced to decelerate the motion in the opposite direction via an eccentric action. An eccentric overload is accomplished by performing the eccentric action over a smaller angular displacement therefore, with a greater muscle torque than the concentric action. Each session consisted of 4 sets of 8 repetitions with 1 minute rest between sets. Additionally, Bourne et al (2017) the observed a 74 and 78% increase in peak eccentric knee flexor torque performing 10 weeks of Nordic hamstring or hip extension exercises. The Nordic hamstring curl involves the athlete kneeling with their ankles fixed by a partner, maintaining an open hip angle they then lower their body as slow as possible towards a prone position. A resistance band may be used to ensure contraction is maintained throughout the whole movement. The mechanisms behind the shift towards producing higher torque values at longer muscle lengths is believed to be as a result of an increase of sarcomeres in series, and a greater muscle stiffness or spring like qualities from the eccentric exercise. Stiffness and stability at longer muscle lengths may therefore prevent active muscle strain and enhance athletic performance (LaStayo et al, 2003).
Tuck Jump Test
Method
To perform the tuck-jump test the athlete begins in the athletic stance position with feet shoulder width apart. The first jump is initiated with a slight crouch downward, while extending the arms backward. They then swing the arms forward while simultaneously jumping vertically, flexing the hips pulling the knees up as high as possible. Thighs should be parallel at the highest point of the jump; the athlete is encouraged to land softly with a toe to mid foot rocker landing. This is repeated continuously for 10 seconds. The test is assessed using a score sheet which checks specific criteria including knee and thigh motion, foot position on landing and plyometric technique. The assessment can then be carried out pre, during and post any intervention to monitor change in technique over time.
Results
Figure 3 shows the athletes assessment following the tuck-jump test. The only area of concern for this athlete is the presence of lower extremity valgus on landing. As previously stated, knee valgus is a significant predictor of ACL injury, therefore should be addressed.
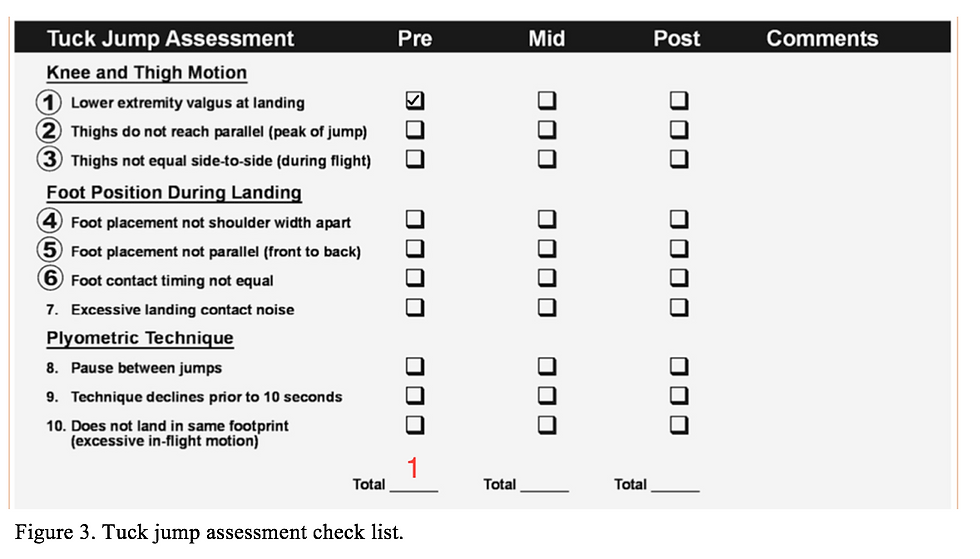
This is further clarified in figure 4 which clearly shows the athlete falling into hip adduction, knee abduction and slight ankle eversion on landing. Pauda et al (2005) reported that hip abduction strength was an important variable in preventing ACL injury as reduced gluteus medius strength resulted in greater knee valgus angles when landing; additionally, landing in knee valgus also correlates with increased ground reaction forces. A preventative program should therefore look at addressing this lack of neuromuscular control. Comprehensive dynamic neuromuscular control programmes that combine strengthening, balance flexibility and plyometric exercise supported with appropriate feedback and technical correction have been shown to effective at improving hip, knee and ankle biomechanics during landing or rebound jumps.
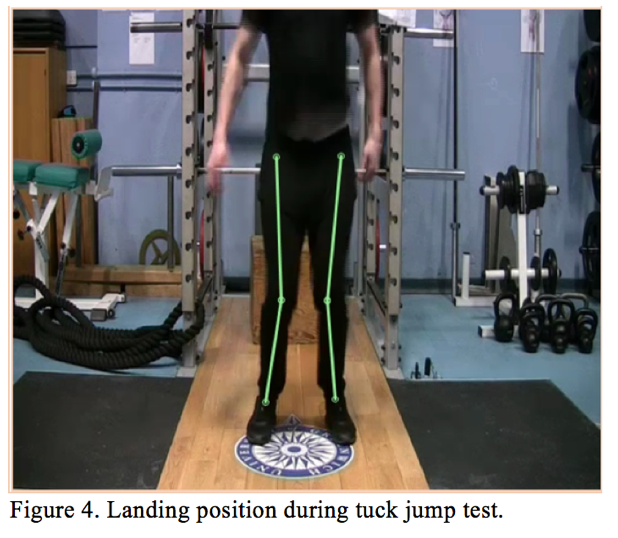
Recommendations
Improve neuromuscular control of landing technique – reduce tuck jump assessment score to zero.
The counter movement jump exercise with a stick and hold can be used initially to develop neuromuscular control on landing. This exercise requires minimal knee flexion and most of the vertical propulsion is developed from ankle plantar flexion. Coaching cues such as ‘keep knees square’, ‘no inward knee bend’ and ‘keep knees apart’ should be used to reinforce proper technique. This first step needs to make the athlete aware of what proper form and technique feels like and the difference from undesired or potentially dangerous positions. Video footage can help provide visual feedback to the athlete to further emphasise these points. Low intensity plyometrics are therefore used to strengthen the landing technique. 180˚ turns can be incorporated to move from the sagittal plane into the transverse plane. This exercise can be progressed into a stop jump, where the athlete performs a broad jump for distance and upon landing, sticks and holds that position for 3-5 seconds. This forces the athlete to maintain dynamic control of the knee over prolonged period of time, and recognise good knee and foot position, enhancing proprioceptive abilities. When knee valgus is observed the coach should provide the cues as mentioned above. A further progression for this may include a drop and stick, where the athlete now drops from a predetermined height (20-30 cm), to land in the athletic position, again receiving verbal feedback where necessary. Once the landing technique is mastered, single leg-hop and holds (with deep knee flexion) can be used, followed by more dynamic exercises that involve the stretch shortening cycle such as single leg hops over hurdles, rebound jumps, drop jumps and single/double leg bounding.
Injury Prevention Program
From the testing process, it is suggested that this athlete increases eccentric strength of the hamstrings and improves neuromuscular control during landing. Two strengthening exercises will be suggested for the hamstring in addition to a progressive jump training programme (figure 5). The objective of the neuromuscular exercises will re-enforce safe landing positions, therefore constant cueing from the coach is required to identify any flaws. These exercises all emphasise a stick at the end of the movement to allow the athlete to experience prolonged dynamic control of the hip, knee and ankle. Once this landing technique is mastered the jumps will become more dynamic; for example, the drop and stick will become a drop jump, and the jump stop will become continuous broad jumps and single leg hops/bounds can be incorporated.
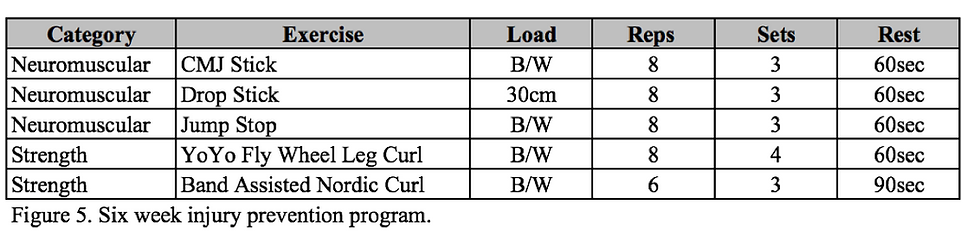
This program will be followed over a six-week period, performed twice per week, with small increments in volume throughout the intervention. Neuromuscular exercises will be progressed according to technical ability. The injury prevention program will be completed after a general warm-up before the commencement of the main session for that day. The six-week intervention allows time to progress the neuromuscular exercises into high intensity plyometrics (i.e. drop jumps, single leg hops/bounds). After the six-week intervention, baseline isokinetic and tuck jump tests will be repeated to indicate effectiveness of the program, with a goal of >0.80 functional Hecc:Qcon anda zero score on the tuck jump assessment.
Conclusion
Both the conventional and functional H:Q ratio should be considered when measuring knee function. The functional H:Q ratio reflects how the agonist and antagonist muscles work around the knee during movement, therefore a deficiency in this ratio (<0.80) may be indicative of injury risk, as this would indicate insufficient eccentric braking efficiency of the hamstring compared to the quadriceps. Eccentric strength training has been shown to result in the hamstring muscles being able to produce higher forces at longer lengths, reducing the incidence of injury. Knee valgus during landing mechanics is strongly correlated with ACL injury risk. Standardised jump landing risk assessments can be used to highlight athletes at risk of ACL injury. Neuromuscular training to correct landing mechanics and progression into high intensity plyometrics can lower the risk of ACL injuries in athletes.
References
Aagaard, P., Simonsen, E. B., Trolle, M., Bangsbo, J., & Klausen, K. (1995). Isokinetic hamstring/quadriceps strength ratio: Influence from joint angular velocity, gravity correction and contraction mode. Acta Physiologica Scandinavica,154(4), 421-427. doi:10.1111/j.1748-1716.1995.tb09927.x
Askling, C., Karlsson, J., & Thorstensson, A. (2005). Hamstring injury occurrence in elite soccer players after preseason strength training with eccentric overload. Scandinavian Journal of Medicine and Science in Sports,15(1), 65-65. doi:10.1111/j.1600-0838.2005.00443.x
Arnason, A., Andersen, T. E., Holme, I., Engebretsen, L., & Bahr, R. (2007). Prevention of hamstring strains in elite soccer: An intervention study. Scandinavian Journal of Medicine & Science in Sports,18(1), 40-48. doi:10.1111/j.1600-0838.2006.00634.x
Bahr, R. (2003). Risk factors for sports injuries -- a methodological approach. British Journal of Sports Medicine,37(5), 384-392. doi:10.1136/bjsm.37.5.384
Baltzopoulos, V., & Brodie, D. (1989). Isokinetic Dynamometry. Sports Medicine,8(2), 101-116. doi:10.2165/00007256-198908020-00003
Bien, D. P. (2011). Rationale and Implementation of Anterior Cruciate Ligament Injury Prevention Warm-Up Programs in Female Athletes. Journal of Strength and Conditioning Research,25(1), 271-285. doi:10.1519/jsc.0b013e3181fb4a5a
Boling, M. C., Padua, D. A., Marshall, S. W., Guskiewicz, K., Pyne, S., & Beutler, A. (2009). A Prospective Investigation of Biomechanical Risk Factors for Patellofemoral Pain Syndrome. The American Journal of Sports Medicine,37(11), 2108-2116. doi:10.1177/0363546509337934
Bourne, M. N., Timmins, R. G., Opar, D. A., Pizzari, T., Ruddy, J. D., Sims, C., Shield, A. J. (2017). An Evidence-Based Framework for Strengthening Exercises to Prevent Hamstring Injury. Sports Medicine,48(2), 251-267. doi:10.1007/s40279-017-0796-x
Cameron M, Adams R, Maher C. (2003) Motor control and strength as predictors of hamstring injury in elite players of Australian football. Phys Ther Spor;4(4):159–66.
Campbell DE, Glenn W. (1982) Rehabilitation of knee extensor and flexor muscle strength in patients with meniscectomies, ligamentous repairs and chondromalacia. Physical Therapy 62: 10-15
Croisier, J., Forthomme, B., Namurois, M., Vanderthommen, M., & Crielaard, J. (2002). Hamstring Muscle Strain Recurrence and Strength Performance Disorders. The American Journal of Sports Medicine,30(2), 199-203. doi:10.1177/03635465020300020901
Croisier JL, Ganteaume S, Binet J, Genty M, Marcel JF. (2008) Strength Imbalances and Prevention of Hamstring Injury in Professional Soccer Players: A Prospective Study. Am J Sports Med;36:1469- 1475.
Drezner, J. A. (2003). Practical Management: Hamstring Muscle Injuries. Clinical Journal of Sport Medicine,13(1), 48-52. doi:10.1097/00042752-200301000-00009
Ekstrand, J., Hagglund, M., & Walden, M. (2009). Injury incidence and injury patterns in professional football: The UEFA injury study. British Journal of Sports Medicine,45(7), 553-558. doi:10.1136/bjsm.2009.060582
Faunø P, Wulff Jakobsen B (2006) Mechanism of anterior cruciate ligament injuries in soccer. Int J Sports Med 27:75-79
Fousekis K, Tsepis E, Poulmedis P. (2011) Intrinsic risk factors of non-contact quadriceps and hamstring strains in soccer: a prospective study of 100 professional players. Br J Sports Med;45(9):709–14.
Gleeson, N., & Mercer, T. (1996). The Utility of Isokinetic Dynamometry in the Assessment of Human Muscle Function. Sports Medicine,21(1), 18-34. doi:10.2165/00007256-199621010-00003
Hewett TE, Stroupe AL, Nance TA, Noyes FR. (1996) Plyometric training in female athletes. Decreased impact forces and increased hamstring torques. Am J Sports Med;24(6):765–773. [PubMed: 8947398]
LaStayo P, Woolf J, Lewek M (2003). Eccentric muscle contrac- tions: their contribution to injury, prevention, rehabilitation, and sport. J Orthop Sports Phys Ther; 33 (10): 557-71
Renstrom, P., Ljungqvist, A., Arendt, E., Beynnon, B., Fukubayashi, T., Garrett, W., Engebretsen, L. (2008). Non-contact ACL injuries in female athletes: An International Olympic Committee current concepts statement. British Journal of Sports Medicine,42(6), 394-412. doi:10.1136/bjsm.2008.048934
Soomro, N., Sanders, R., Hackett, D., Hubka, T., Ebrahimi, S., Freeston, J., & Cobley, S. (2016). The Efficacy of Injury Prevention Programs in Adolescent Team Sports. The American Journal of Sports Medicine,44(9), 2415-2424. doi:10.1177/0363546515618372
van Mechelen W. Sports injury surveillance systems. ‘One size fits all’? (1997)Sports Med.;24(3):164–168.