
Nutrition, training and overall health are important aspects of successful sport performance. Optimising nutritional intake can improve and accelerate recovery, achieve and maintain an optimal body weight and minimise the risk of injury and illness. To optimise training and performance through nutrition it is essential to ensure the athlete is consuming enough calories to offset energy expenditure (Berning 1998). A negative energy balance often occurs in endurance sports such as cycling and triathlon as well as sports in which dietary restriction is a strategy to modify body composition rapidly such as gymnastics, dancing, wrestling and Judo (Kreida et al, 2004). The latter example often results in athletes attempting to lose weight too quickly and in mismanaged ways (Artioli et al, 2010). As a result, training with an energy deficient diet will lead to significant weight/muscle mass loss, illness, symptoms of overtraining and reductions in performance (Kreida 1998).
For team sport athletes such as football players, the energy expenditure will vary depending on position, playing style and game strategy (Gibala 2013). There may also be a need for change in body mass and composition, often requiring increased muscle mass and sometimes reduced body fat (Maughan 2006). A nutritional plan should therefore follow an individualised approach taking into consideration age, gender, body composition, environmental training conditions, genetics and duration, frequency and intensity of training (Baechle & Earle 2008).
The aim of this report is to identify different nutritional strategies available to the football player and the impact they may have on performance. This will be followed by a review of the basic physiology and energy requirements of football, then finally a case study suggesting nutritional strategies to optimise a players’ performance.
Nutritional Strategies
Train Low – Compete High
This eating strategy requires the athlete to train with low glycogen/carbohydrate availability to enhance the training response, but compete with high fuel availability to promote performance (Burke 2010). Hansen et al (2005) observed an 85% increase in time to exhaustion after 10 weeks of training in a glycogen depleted state. The mechanism behind the increase was thought to be an increase in citrate synthase (CS) and 3-hydroxyacyl-CoA (HAD) which are important enzymes in fat metabolism, therefore increasing the capacity to use fat as a fuel during exercise. Strategies to reduce carbohydrate availability include exercising after an overnight fast, consuming water during prolonged training sessions, withholding carbohydrate in the hours after exercise and restricting carbohydrates below the fuel requirements of the training load (Burke 2010). Although this maybe an effective strategy for endurance based athletes, it may not be suited to the football player due to the possible impairment of the ability to train at high intensities as there appears to be little positive effect on exercise performed at high intensity. This is due to the down regulation of the enzymes that oxidise carbohydrates, therefore reducing the ability of the muscle to provide energy quickly (Burke 2007).
Ketogenic Diet
An extreme dietary restriction of carbohydrate (e.g. less than 40-50g per day) will result in a metabolic state of ketosis. Fat consumption is often high in a ketogenic diet with moderate levels of protein intake. In this state, whole body metabolism gradually shifts to oxidising a greater amount of energy from fat stores. The high rates of lipolysis will lead to increased amounts of acetyl-coenzyme A and the formation of ketone bodies in the mitochondrial matrix of the liver (Antonio 2008). Ketone production is therefore an indication that lipolysis has been accelerated and that all enzymes involved in fat metabolism are activated. A major limitation of the studies looking into ketogenic diets on athletic performance is the inadequate time allowed for the body to fully adapt to the diet. Most studies investigating the dietary effects on exercise last for a few days or at most a week, whereas it may take several weeks to enter a state of ketosis. When fully adapted to this diet, there appears to be no decrement in submaximal endurance capacity, but again may not be suitable for high intensity exercise (Antonio 2008). This type of nutritional strategy may be more suited to individuals presenting with metabolic syndrome, risk of diabetes and cardiovascular disease, as these populations tend to exercise at a lower intensity. Volek et al (2002) showed the aesthetic benefits of a ketogenic diet in maximising fat loss while maintaining lean body mass. This may be an advantage in sports which have an emphasis on body composition.
Vegan/Vegetarian Diet
Veganism is a form of vegetarianism which prohibits the consumption of any animal based products and is normally adopted on the basis of strong ethical beliefs based on the welfare of animals. Due to the elimination of all animal products a poorly constructed vegan diet may result in both macronutrient (protein) and micronutrient (vitamin B12, vitamin D, iron, zinc, calcium and iodine) deficiencies. Protein deficiency for the athlete is a major concern as protein serves as a substrate for exercise performance and also a catalyst for exercise adaptation (Tipton 2004). The International Society of Sports Nutrition suggest a protein intake of 1.4-2.0 g×kg×day (Campbell et al 2007) as appropriate for most athletes, but recommendations of up to 1.8-2.7 g×kg×day are suggested for vegan athletes due to decrease digestibility and absorption of plant based protein sources (Phillips 2011). As plant based proteins also often lack the essential amino acids, it is also recommended that a wide range of plant based proteins are consumed in order to support recovery and adaptation to training. This includes foods such as grains, legumes, nuts and seeds are consumed. The use of plant based protein powders (e.g. soy, pea) may also help with dietary supplementation and aid in the recovery of training (Joy et al 2013).
Ramadan
Ramadan is a month long religious festival whereby Muslims refrain from eating, drinking, smoking and having sexual relations from sunrise to sunset. Muslims are often required to continue training during Ramadan intermittent fasting in order to be prepared for competition. Meckel et al (2008) showed significant decrease in aerobic capacity during Ramadan, likewise Hamouda et al (2011) observed a significant decrease in Yo-Yo intermittent recovery test performance in young football players. Concerning anaerobic performance, Chtourou et al (2011) found a decrease in peak and average power output during a Wingate test on a cycle ergometer and a decrease in repeated sprint ability time during Ramadan intermittent fasting. This was thought to be as a result of decreased glycolytic capacity and slower creatine phosphate replenishment during rest periods of the sprints. Athletes therefore need to manage training program variables properly during Ramadan which means manipulating the intensity, frequency and volume of sessions during this period. This was shown by Rebaï et al (2013) who observed a significant increase in muscle strength and power of young football players when a using tapering period characterised by reduction in training volume during Ramadan intermittent fasting.
Carbohydrate Loading
This strategy refers to the practice of maximising or supercompensating muscle glycogen stores prior to athletic performance. The rationale behind this is that endurance or capacity to perform prolonged moderate intensity work is determined by pre-exercise levels of muscle glycogen (Hawley et al 1997). The classical 7-day carbohydrate loading model consists of 3-4 days of glycogen depletion through strenuous exercise followed by a 3-4 day, high loading phase which includes a taper in exercise volume/intensity. However, well trained athletes are able to show supercompensation of muscle glycogen without the initial depletion phase and a loading phase of 36-48 hours when carbohydrates equate to 7-10 g×kg within the diet (Bussau et al 2002). Team sports that involve intermittent high intensity exercise are associated with high rates of glycogen utilisation, and potential depletion during the event. Therefore, performance in these types of sports may benefit from supercompensation of muscle glycogen stores. A problem may arise in sports such as football when teams often play twice within the same week. This could prevent full carbohydrate loading in preparation for the second match.
Physiology of Football
The overriding characteristic of football is the pattern of intermittent activity between repeated bouts of high intensity play interspersed with low to moderate active recovery or rest. During a 90-minute match players can cover between 10-12km (Di Salvo 2008), with high intensity actions (e.g. high speed running and sprinting) accounting for approximately 8-10% of total distance (Rampinini et al 2007). High aerobic fitness is therefore essential in order for football players to sustain intense exercise for long durations and recover more rapidly between high intensity phases of the game (Iaina et al 2009). Although high intensity actions make up a relatively low percentage of match distance, these actions must not be underestimated as they are critical in deciding the outcome of the competition. Elite players can perform between 150-250 brief intense actions (Mohr et al 2003) and the most extreme periods can require up to five high intensity actions within one minute (Carling et al 2012), which indicates a high rate of anaerobic energy turnover at certain times. However, the activity profiles of match play can vary depending on player position, formation, tactics employed, level of opposition and playing surface (Di Salvo et al 2013, Bradley et al 2013, Rampinini et al 2007, Anderson et al 2008).
The aerobic energy system contributes significantly to the energy produced during intermittent exercise throughout both the exercise and rest periods, whereas the anaerobic systems provide energy during the exercise bouts (Garrett & Kirkendall 2000). It has been shown that both the phosphagen and anaerobic glycolysis energy pathways are activated simultaneously during brief high intensity bursts of activity, indicating both muscle glycogen and creatine phosphate being the primary substrates (Spencer et al 2005). Creatine phosphate to some extend can be re-synthesised during the following low intensity period (Bangsbo 1994) but could drop to less than 30% of resting values during periods of the game of multiple high intensity actions with little rest in between (Bangsbo et al 2006). During the recovery periods fat oxidation can also be substantial with glucose taken up from the blood being another major substrate (Garrett & Kirkendall 2000). The high blood lactate levels of up to 12 mmol×1-1 observed during matches (Bangsbo et al 2006) are evidence of increased rates of glycolysis. These high values are understood to be as a result of an accumulation of high intensity bouts, rather than a single action during the game (Bangsbo et al 2006). The high reliance on both the aerobic (powered by carbohydrate and fats) and the anaerobic systems (powered by phophagen and anaerobic glycolysis) highlight carbohydrate as a dietary priority because it is a fuel for both energy systems (Burke et al 2006). Additionally, Bangsbo et al (2006) observed an association with decreased sprint performance and depleted muscle glycogen stores, further indicating the importance of elevated glycogen stores in high intensity performance.
Energy Requirements
During a normal training week, a player will undertake individual and team based sessions consisting of endurance, speed, strength, tactical and technical drills and match play (Bangsbo et al 2006). The nature, volume and intensity of training will vary depending on the period of the season, for example players may be required to train twice a day during pre-season resulting in an increase in the training load; energy intake must therefore be increased to meet this demand. During the season, weekly training load displays evidence of periodisation which appears to depend on the proximity of the match (Anderson et al 2015) and also the number of matches scheduled for that week (Morgans et al 2014).
Creating an energy balance requires the direct measurement of energy in and energy expended. The energy expenditure and requirements of football players arise from the contribution of basal metabolic rate, thermic effect of food, thermic effect of activity and in some cases growth (Manore & Thompson 2006). Given the fluctuations in daily training load it would appear that energy expenditure of football players may vary accordingly throughout the training week, therefore requiring energy intake to be adjusted for that particular day (Anderson et al 2017). Measuring energy expenditure is a complex process and will vary on the method used; a method commonly used for athletes is the doubly labelled water method. Anderson et al (2017) used this method to measure energy expenditure of elite football players over a seven-day training week which included two competitive matches. They found the average energy expenditure to be 3566 ± 585 kcal per day, with a higher carbohydrate consumption on match days (6.4 ± 2.2 g×kg-1) compared to training days (4.2 ± 1.4 g×kg-1). Another less expensive method available is through the use of equations. Thompson and Manone (1996) found that for both active males and females the Cunningham equation was the best predictor (see case study). This equation uses the lean body mass value of the athlete, which is multiplied by weighted factor to represent activity levels. Once an estimate on energy expenditure is established, energy intake can be assessed with the use of self-reported dietary logs. All of this information can then be collated to establish whether the athlete is in an energy balance.
Nutritional Recommendations in Football
Macronutrient intake in football should follow a periodised approach due to the day to day fluctuations in training load, and specific periods of the season (e.g. carbohydrate intake should be higher in the pre-season to match the increased training load-training twice a day on some occasions). When training intensity drops then so should carbohydrate consumption. Protein may vary from 1.4-2.0 g×kg×day but may increase during periods of higher intensity training (Campbell et al 2007). Fat consumption typically remains unchanged, although may be manipulated to alter energy balance when required. Football players must include strategies to fuel and re fuel between matches and training. The following carbohydrate recommendations have been suggested from Burke, Loucks and Broad (2006)
Low intensity training day = 5-7 g×kg
Moderate to high intensity day = 7-10 g×kg
Day before competition to increase glycogen stores = 8-10 g×kg
Case Study
Sport: Football
Position: Centre Midfield
Age: 27
Body Weight: 77 kg
Body Fat%: 11.0%
LBM: 68 kg
Height: 180 cm
Introduction
This professional player trains 3-4 days and can play 1-2 competitive matches within the training microcycle. The body fat percentage of this player is adequate in regard to the position he plays (Sutton et al 2009), therefore the objective of this dietary intervention is to maintain body weight, whilst providing sufficient energy to cope with the demands of typical training week consisting of 4 training days and 1 competitive match.
Establishing Energy Requirements
Burke et al (2015) suggest the Cunningham equation to best predict energy requirements using an equation. This involves using the athletes lean body mass to estimate resting metabolic rate, then multiply this by weighted factor that represents the physical activity level (PAL) of the individual.
RMR (kcal×day) = 500 + 22 (LBM)
RMR (kcal×day) = 500 + 22 (68)
1996 = 500 + 22 (68)
1996 x PAL = Energy Expenditure (kcal×day)
1996 x 2.1 = 4191 kcal×day (Heavy day)
1996 x 1.6 = 3193 kcal×day (Light Day)
Establishing Energy Intake
On completion of a 3-day diet diary, the players energy intake was estimated. It was observed that the player was in a negative energy balance which could lead to weight loss, loss of muscle mass and potential illness if continued along with the high training loads.
Calculating Macronutrients
Traditionally, macronutrient intake has been expressed as percentage of total energy intake, however this can be a confounding variable that may result in misleading values in relation to absolute macronutrient intake. Therefore, it is suggested that these values are expressed as grams per kg of body weight (g×kg). With 1g of carbohydrate and protein equal to 4 kcal and 1g of fat equal to 9 kcal, daily energy intake can be calculated.
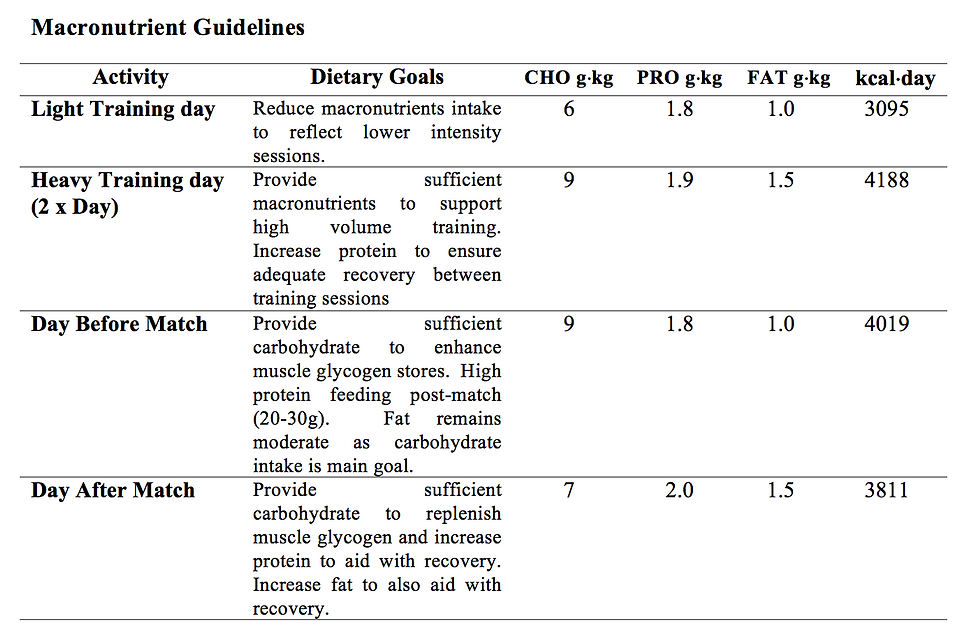
Example Day
This example is for a typical day following a match.
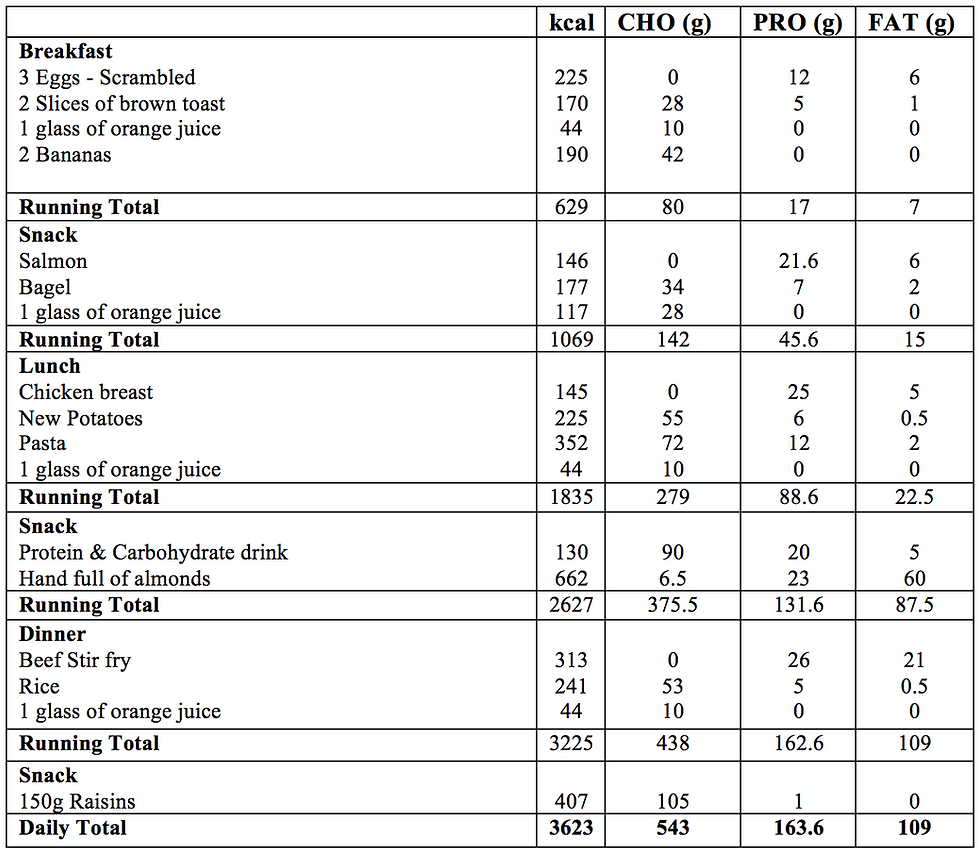
Conclusion
Football is a sport characterised by intermittent periods of high intensity separated by periods of low intensity activity or rest. Maintaining an energy balance that ensures the athlete receives adequate macronutrient intake to fuel performance and training is essential in maintaining high levels of performance and to recover sufficiently. The oscillation of training volume and intensity will effect both energy requirements and metabolism, therefore a periodised approach to nutrition which alters macronutrient intake in relation to the training and competition schedule is the most effective approach. In order to perform multiple bouts of high intensity activity and facilitate speedy recovery for the next match, it is essential to ensure muscle glycogen stores are fully stored and quickly replenished post-match.
References
Anderson, L., Orme, P., Di Michele, R., Close, G.L., Morgans, R., Drust, B., & Morton, J.P. (2015). Quantification of training load during one-, two- and three-game week schedules in professional soccer players from the English Premier League: implications for carbohydrate periodization. J Sports Sci, 4, 1- 10.
Anderson, L., Orme, P., Naughton, R. J., Close, G. L., Milsom, J., Rydings, D., . . . Morton, J. P. (2017). Energy Intake and Expenditure of Professional Soccer Players of the English Premier League: Evidence of Carbohydrate Periodization. International Journal of Sport Nutrition and Exercise Metabolism, 27(3), 228-238.
Antonio, J. (2008). Essentials of sports nutrition and supplements. Totowa, NJ: Humana Press.
Artioli, G. G., Franchini, E., Nicastro, H., Sterkowicz, S., Solis, M. Y., & Lancha, A. H. (2010). The need of a weight management control program in judo: a proposal based on the successful case of wrestling. Journal of the International Society of Sports Nutrition, 7(1), 15.
Baechle, T. R., & Earle, R. W. (2008). Essentials of strength training and conditioning. Champaign: Human Kinetics.
Bangsbo, J., Mohr, M., & Krustrup, P. (2006). Physical and metabolic demands of training and match-play in the elite football player. Journal of Sports Sciences, 24(7), 665-674. doi:10.1080/02640410500482529
Bangsbo, J. (1994) The physiology of soccer - With special reference to intense intermittent exercise. Acta Physiologica Scandinavica, 151(S619), 84-106. doi:10.1111/j.1365-201x.1994.tb10650.x
Bradley, P.S, and Noakes T.D. (2013) Match running performance fluctuations in elite soccer: Indications of fatigue pacing or situation influence? Journal of Sport Sciences. 31 (15): 1627-1638
Burke, L., Loucks, B., and Broad, N. (2006) Energy and carbohydrate for training and recovery. Journal of Sports Science 24 :675-685
Burke, L. M. (2010). Fueling strategies to optimize performance: training high or training low? Scandinavian Journal of Medicine & Science in Sports, 20, 48-58
Burke, L., Deakin, V., & Allanson, B. (2015). Clinical sports nutrition. North Ryde, N.S.W., Australia: McGraw-Hill Education (Australia) Pty Ltd.
Burke, Louise M. M. "Nutrition Strategies for the Marathon: Fuel for Training and
Racing." Sports medicine 37.4-5 (2007): 344-7.
Bussau, V., Fairchild, T., Rao, A., Steele, P., & Fournier, P. (2002). Carbohydrate loading in human muscle: an improved 1 day protocol. European Journal of Applied Physiology, 87(3), 290-295. doi:10.1007/s00421-002-0621-5
Campbell B, Kreider RB, Ziegenfuss T, La Bounty P, Roberts M, Burke D. (2007) International Society of Sports Nutrition position stand: protein and exercise. J Int Soc Sports Nutr.;4:8.
Chtourou, H., Hammouda, O., Souissi, H., Chamari, K., Chaouachi, A., & Souissi, N. (2011). The Effect of Ramadan Fasting on Physical Performances, Mood State and Perceived Exertion in Young Footballers. Asian Journal of Sports Medicine, 2(3). doi:10.5812/asjsm.34757
Di Salvo, V., Pigozzi, F., Gonzalez-Haro, C., Lauglin, M.S., and De Witt, J.k. (2013) Match performance comparison in top English soccer leagues. International Journal of Sports Medicine. 34(6): 526-532
Garrett, W. E., & Kirkendall, D. T. (2000). Exercise and sport science. Philadelphia, PA: Lippincott Williams & Wilkins.
Gibala, M. J. (2013). Nutritional Strategies to Support Adaptation to High-Intensity Interval Training in Team Sports. Nutritional Coaching Strategy to Modulate Training Efficiency Nestlé Nutrition Institute Workshop Series, 41-49. doi:10.1159/000345817
Hansen AK, Fischer CP, Plomgaard P, Andersen JL, Saltin B & Pedersen BK. (2005). Skeletal muscle adaptation: training twice every second day vs. training once daily. J Appl Physiol 98, 93-99.
Hamouda O, Chtourou H, Farjallah MA. (2011) The effect of Ramadan fasting on the diurnal variations in aerobic and anaerobic performances in Tunisian youth soccer players. Biol Rhythms Res;1:1-15.
Hawley, J.A., Schabort, E.J., Noakes, T.D. et al. Sports Med. (1997) 24: 73.
Iaia, F.M., Rampinini, E., and Bangsbo, J. (2009) High intensity training infootball. International Journal of Sports Physiology and Performance 4(3): 209-306
Joy JM, Lowery RP, Wilson JM, Purpura M, De Souza EO, Wilson SM. (2013) The effects of 8 weeks of whey or rice protein supplementation on body composition and exercise performance. Nutr J;12(1):86.
Kreider RB, Fry AC, O'Toole ML. (1998) Overtraining in Sport. Champaign, IL.: Human Kinetics Publishers.
Manore, M., & Thompson, J. (2006). Energy requirements of the athlete: Assessment and evidence of energy efficiency. In L. Burke & V. Deakin (Eds.), Clinical sports nutrition (3rd edn., pp. 113 – 134). Sydney, NSW: McGraw-Hill
Maughan RJ, (2006) ed: Nutrition and Football. London: Routledge.